2.1
Analysis of solid-liquid interval determination and phase
The DTA curve of
ADC12 aluminum alloy is shown in Figure 4. A red area can be observed, which is the endothermic peak, that is, the Al-Si binary reaction. This area is the solid-liquid interval. The alloy starts to melt at 539°C, completely melts at 578°C, and solidifies at 590°C, so the solid-liquid interval is between 539 and 590°C.
Figure 4 The DTA curve of ADC12 aluminum alloys
The XRD of castings at different sampling positions is shown in Figure 5. The picture shows that it is mainly composed of three phases such as Al, Si and Al2Cu. For the ADC12 aluminum alloy, the α-Al phase and the eutectic phase of α-Al+Si are crystallized initially, and because the Cu content in the alloy is high, a L→α-Al+Si+Al2Cu eutectic reaction will occur; the final structure of the alloy is mainly composed of three phases such asα-Al, Si, and Al2Cu.
Figure 5 XRD of ADC12 aluminum alloys at different sampling locations
2.2
Microstructure and formation mechanism
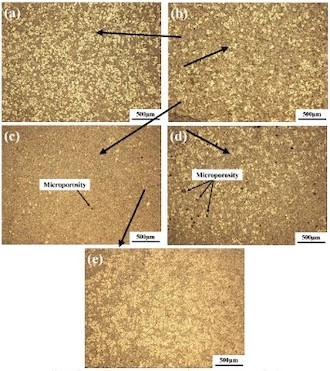
Figure 6 Metallographic structure at different positions under a 50x optical microscope
Figure 6 shows the metallographic structure at different positions. It can be seen that the structure of each position of the gating system is significantly different. A and B have more uniform and rounded primary α-Al, and C and D have less primary α-Al content. The primary α-Al at the nearest handle E of the chamber is in the form of white coarse dendrites, and the solid phase rate is the highest. According to the analysis, the reason for this difference in structure is mainly the influence of the cooling rate and filling process. The pressure chamber temperature is 560 to 570℃, while the mold preheating temperature is 200℃. The closer it is to the pressure chamber, the smaller the cooling rate becomes. The handle E is closest to the pressure chamber, has the greatest thickness, and the slowest cooling rate, so the α-Al at E is in the form of coarse dendrites.
Because the size of the gate connecting D at E is small, it hinders the flow of the primary thick dendrites, and only the small primary α-Al and liquid phase can pass through, so the solid phase at E is the most. As the filling distance increases, the cooling rate at position D to position A gradually increases; the total number of white primary α-Al grains increases, and the content of primary α-Al at position C is the least. This is inconsistent with the phenomenon that the farther away from the pressure chamber is, the less solid content becomes in the research of Li Ming and others. The reason is that, on the one hand, the liquid phase in the slurry in the filling process entangles the fine primary α-Al grains to produce liquid phase segregation, so that there are more solid phases at A and B, and less solid phase at C and D; on the other hand, the liquid phase nucleation grows into fine and round primary α-Al grains due to the high cooling rate at A and B.
In addition, it can also be observed from Figure 6 that there are some obvious black holes in the tissue, which are micropores. In the die-casting process, the alloy slurry fills the mold cavity at high speed, so that the gas that is not discharged in time in the mold cavity is entrained by the alloy melt, and as the alloy melt solidifies, it remains in the test piece to form air holes, that is, black holes. In addition, the microporosity may also be caused by the formation of metal inclusions in the alloy melting process.
Figure 7 shows the metallographic structure at different positions under a high-power microscope. It can be observed that the coarse dendrites in the tissue disappear and evolve into primary α-Al of different sizes and shapes, and the main forms are spherical, nearly spherical, and broken dendrites. In Figure 7e, the primary α-Al has a coarse dendrite morphology, which is consistent with Figure 6e. The primary α-Al observed in Figures 7a and 7d can be generally divided into three types: one is large spherical and nearly spherical particles, labeled as α1-Al; the other is smaller spherical or nearly spherical particles, marked as α2-Al; in addition to these two types, there are the last solidified fine dendrites, marked as α3-Al. Among them, α1-Al and α2-Al are numerous in Figures 7a and 7b, but are rarely distributed in Figures 7c and 7d, mainly fine dendritic α3-Al.
Figure 7 Metallographic structure at different positions under a magnification of 200x
At present, there are two mechanisms for the formation of semi-solid microstructures: one is that the dendrites are broken under the action of external force, and the broken dendrites eventually evolve into spherical grains. A large number of crystal nuclei are produced, and these crystal nuclei inhibit the growth of dendrites and grow directly in a spherical shape. The alloy melt is chilled by the inner wall of the inclined plate, so that a large number of primary α-Al crystal nuclei are generated in the melt close to the inner wall. These crystal nuclei enter the die-cast machine with the melt and fill the mold. Continue to grow, and finally grow into spherical or nearly spherical α1-Al or α2-Al. In addition, the alloy melt flows downward under the action of gravity on the inclined plate, forming a shearing effect on the melt. The dendrites in the melt are sheared and broken to form broken dendrite arms, and at the same time, these broken and fine dendrite arms are preserved due to the chilling effect of the inclined plate.
In the die-casting process, the melt flowing into the pressure chamber from the inclined plate is subjected to the dual effects of chilling and high pressure during injection filling. On the one hand, many primary α-Al crystal nuclei formed by flowing through the inclined plate continue to grow into spherical or nearly spherical α1-Al or α2-Al; on the other hand, under high pressure and high speed, an effect similar to strong stirring is produced on the melt, and the remaining dendrites in the melt continue to be sheared and destroyed. The dendrite arms eventually solidify into spherical or near-spherical grains. However, the cooling speed in the die-casting process is fast, position E is the closest to the pressure chamber due to the different cooling rates at each position, and the cooling speed is the slowest. The dendrites can grow, while the dendrites at positions A to D are too late to grow due to the fast cooling speed, which is solidified into fine and uniform α3-Al. To sum up, rheological die casting with low superheat inclined plates can destroy the growth of dendrites in the melt, and the primary α-Al eventually grows into spherical or nearly spherical grains.